Relative Wind
Knowledge of relative wind is essential for an understanding of aerodynamics and its practical flight application for the pilot. Relative wind is airflow relative to an airfoil. Movement of an airfoil through the air creates relative wind. Relative wind moves in a parallel but opposite direction to movement of the airfoil. [Figure 1]
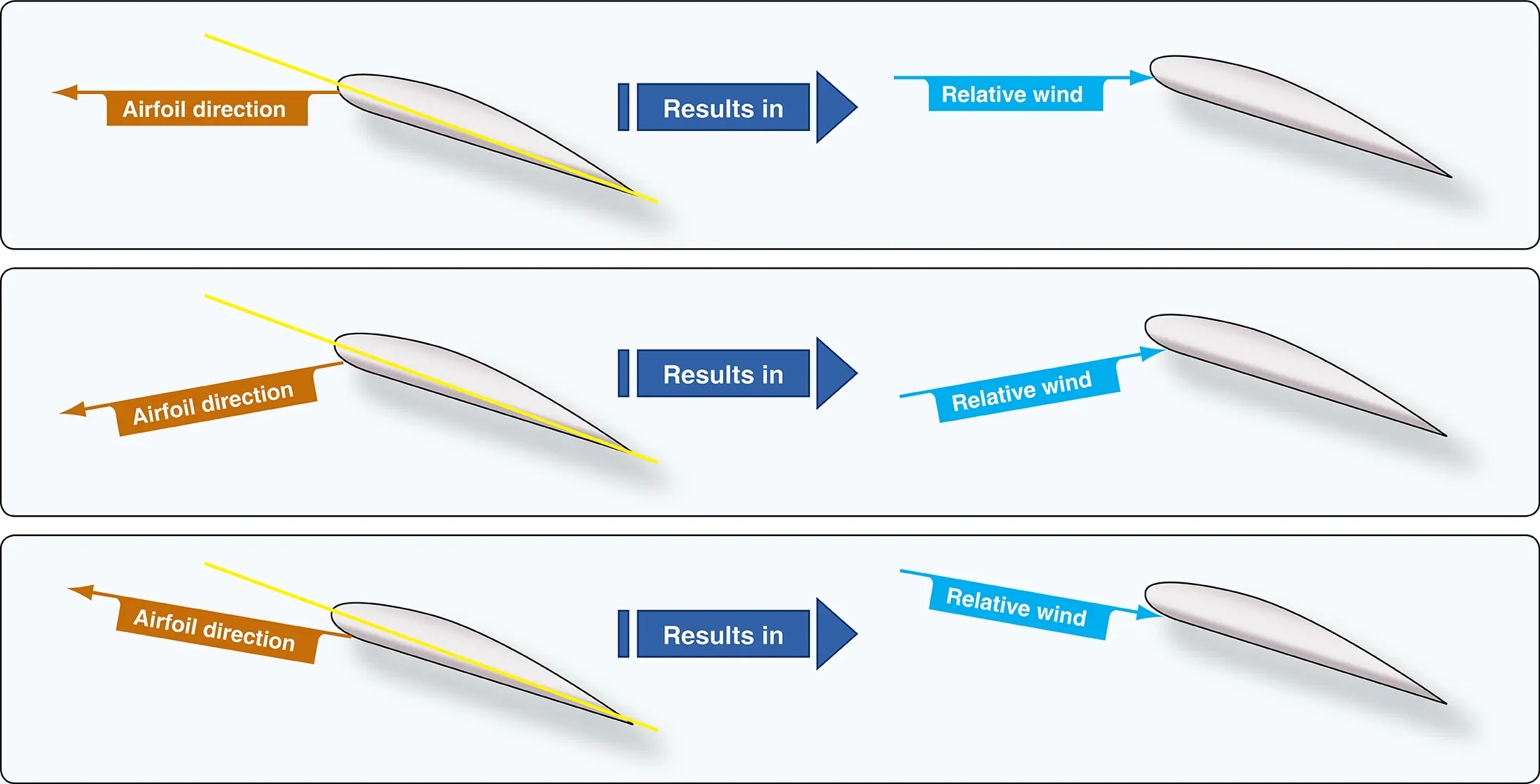
There are two parts to wind passing a rotor blade:
- Horizontal part—caused by the blades turning plus movement of the helicopter through the air [Figure 2]
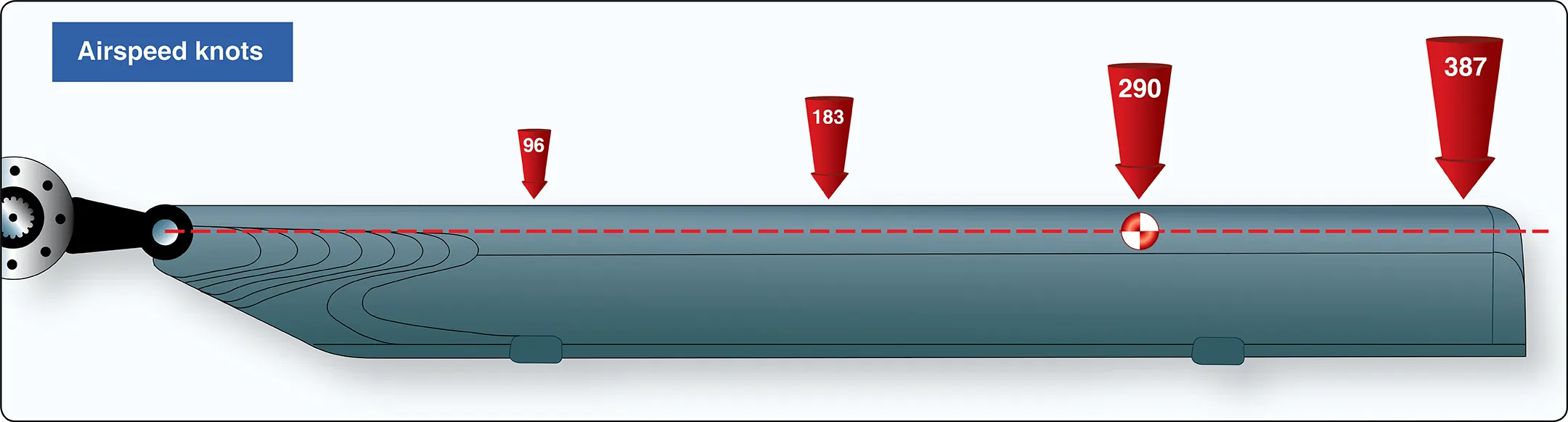
- Vertical part—caused by the air being forced down through the rotor blades plus any movement of the air relative to the blades caused by the helicopter climbing or descending [Figures 3 and 4]
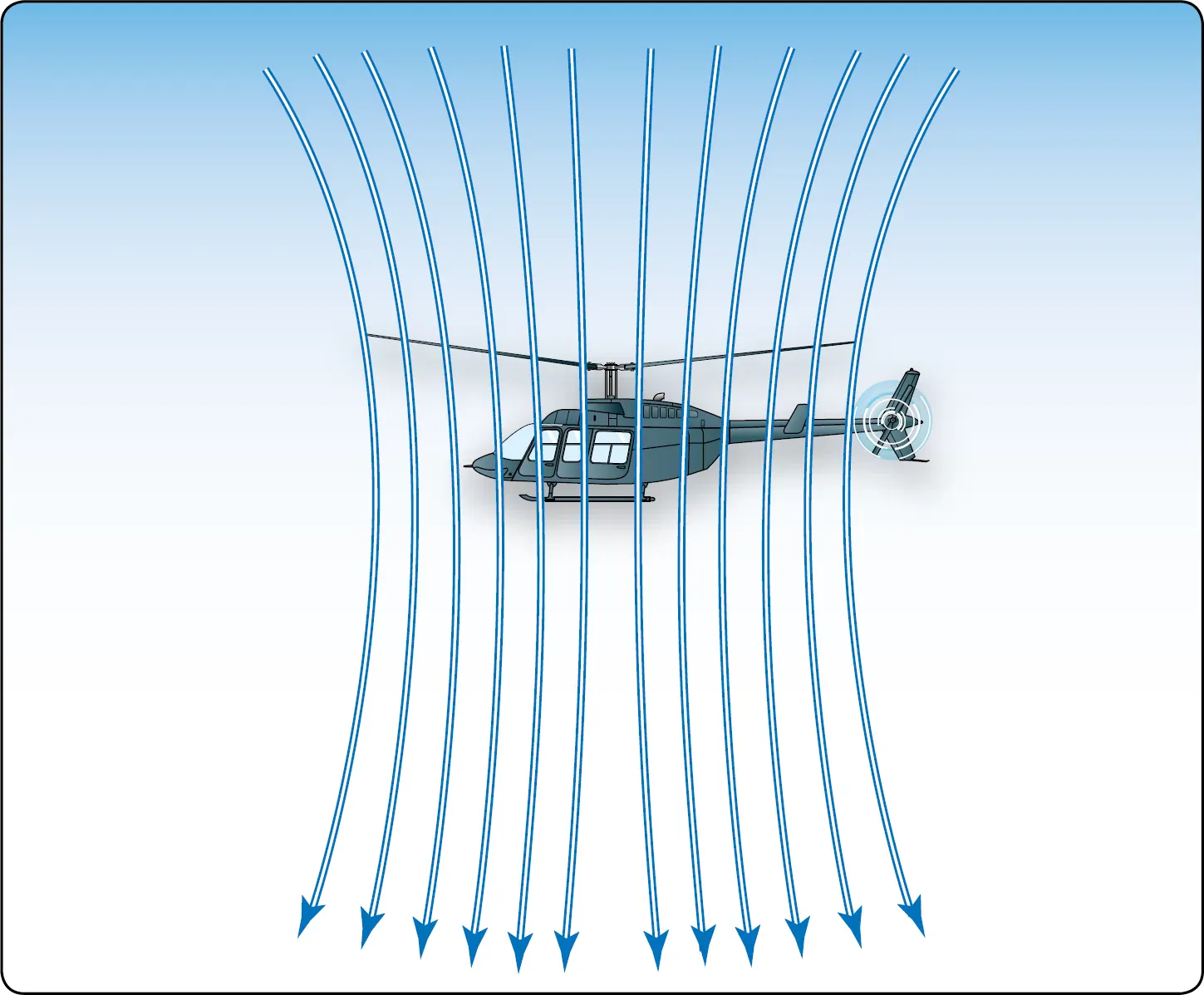
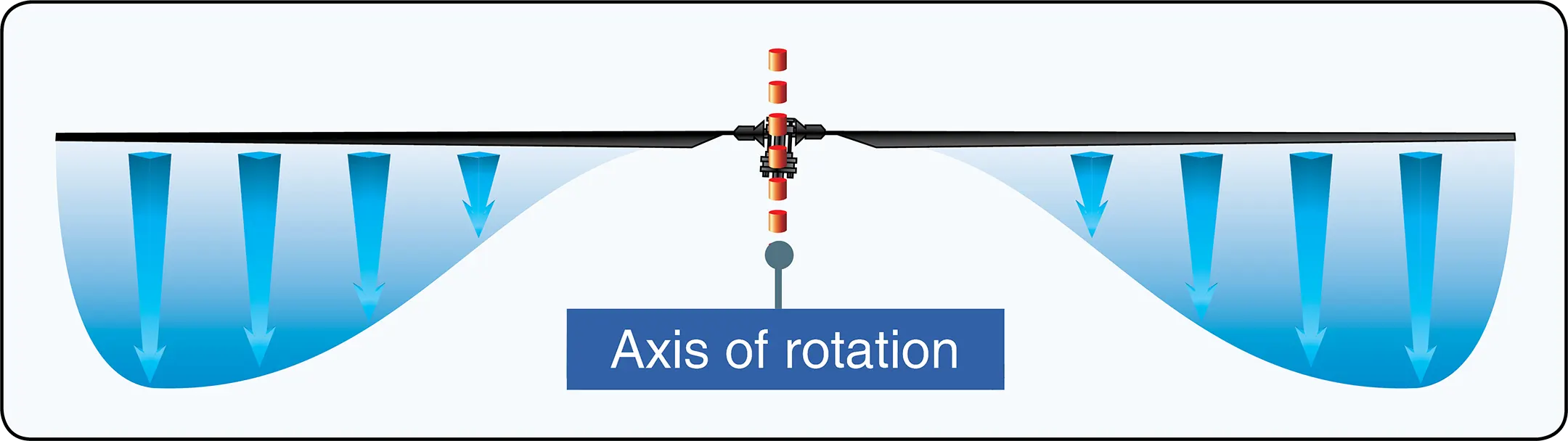
Rotational Relative Wind (Tip-Path Plane)
The rotation of rotor blades as they turn about the mast produces rotational relative wind (tip-path plane). The term rotational refers to the method of producing relative wind. Rotational relative wind flows opposite the physical flightpath of the airfoil, striking the blade at 90° to the leading edge and parallel to the plane of rotation; and it is constantly changing in direction during rotation. Rotational relative wind velocity is highest at blade tips, decreasing uniformly to zero at the axis of rotation (center of the mast). [Figure 5]
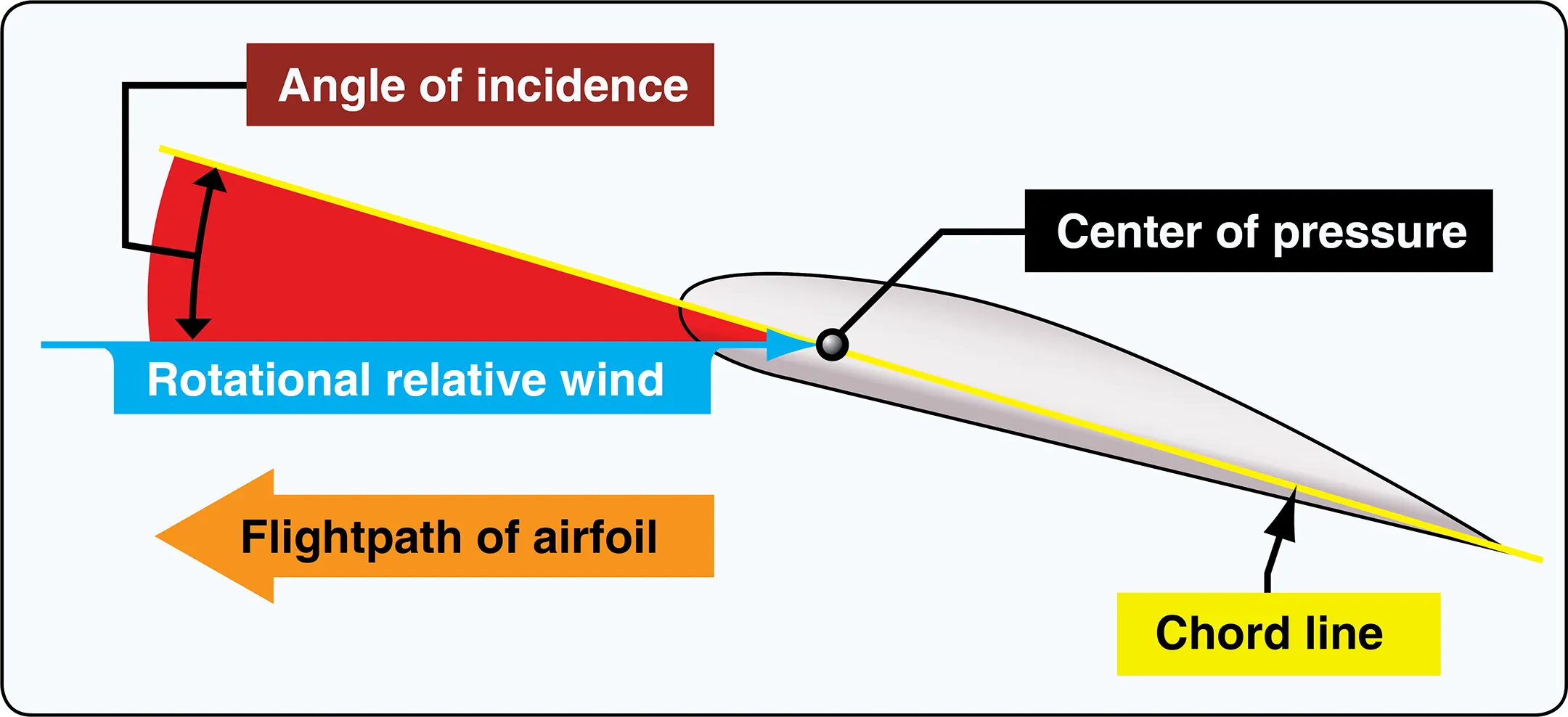
Resultant Relative Wind
The resultant relative wind at a hover is rotational relative wind modified by induced flow. This is inclined downward at some angle and opposite the effective flightpath of the airfoil, rather than the physical flightpath (rotational relative wind). The resultant relative wind also serves as the reference plane for development of lift, drag, and total aerodynamic force (TAF) vectors on the airfoil. [Figure 6]
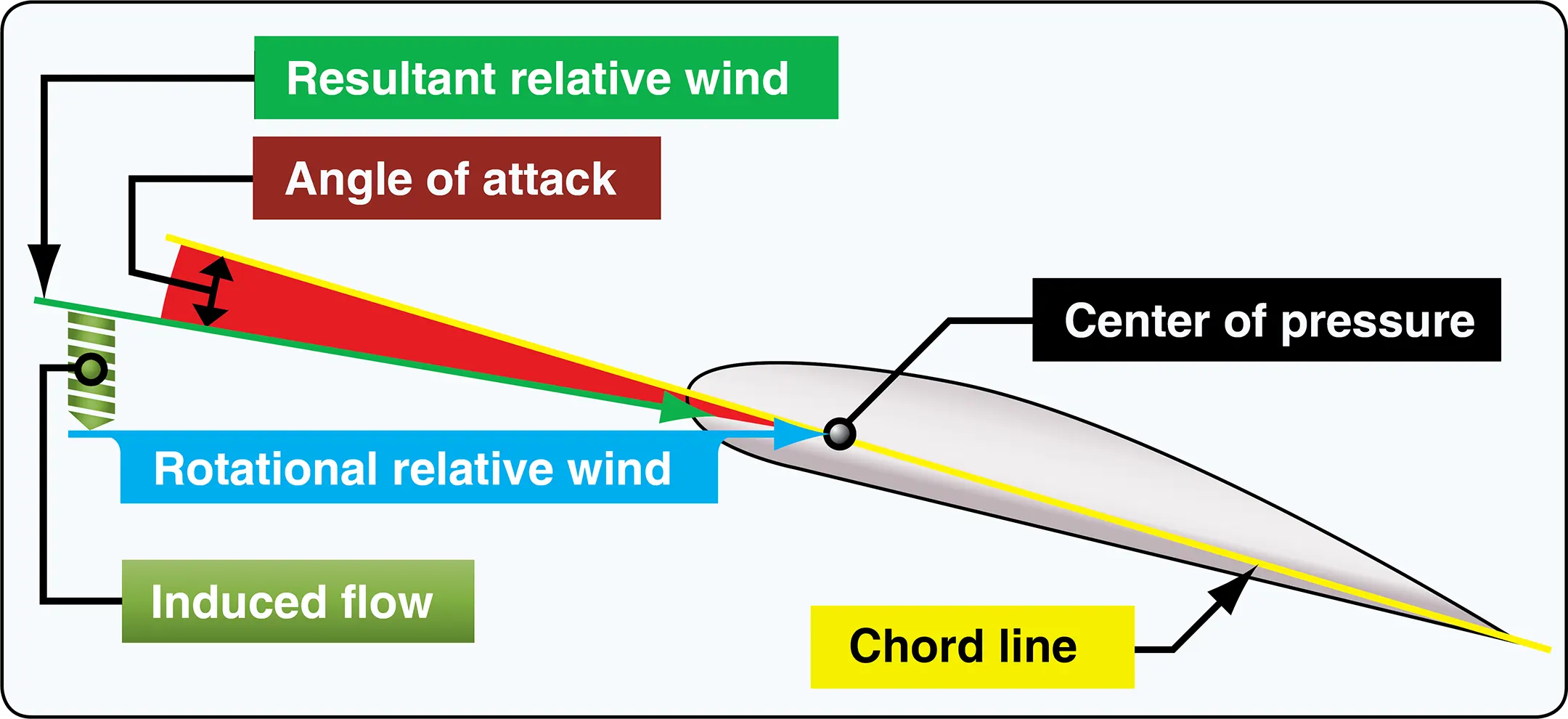
When the helicopter has horizontal motion, airspeed further modifies the resultant relative wind. The airspeed component of relative wind results from the helicopter moving through the air. This airspeed component is added to, or subtracted from, the rotational relative wind depending on whether the blade is advancing or retreating in relation to helicopter movement. Introduction of airspeed relative wind also modifies induced flow. Generally, the downward velocity of induced flow is reduced. The pattern of air circulation through the disk changes when the aircraft has horizontal motion. As the helicopter gains airspeed, the addition of forward velocity results in decreased induced flow velocity. This change results in an improved efficiency (additional lift) being produced from a given blade pitch setting.
Induced Flow (Downwash)
At flat pitch, air leaves the trailing edge of the rotor blade in the same direction it moved across the leading edge; no lift or induced flow is being produced. As blade pitch angle is increased, the rotor system induces a downward flow of air through the rotor blades creating a downward component of air that is added to the rotational relative wind. Because the blades are moving horizontally, some of the air is displaced downward. The blades travel along the same path and pass a given point in rapid succession. Rotor blade action changes the still air to a column of descending air. Therefore, each blade has a decreased AOA due to the downwash. This downward flow of air is called induced flow (downwash). It is most pronounced at a hover under no-wind conditions. [Figure 7]
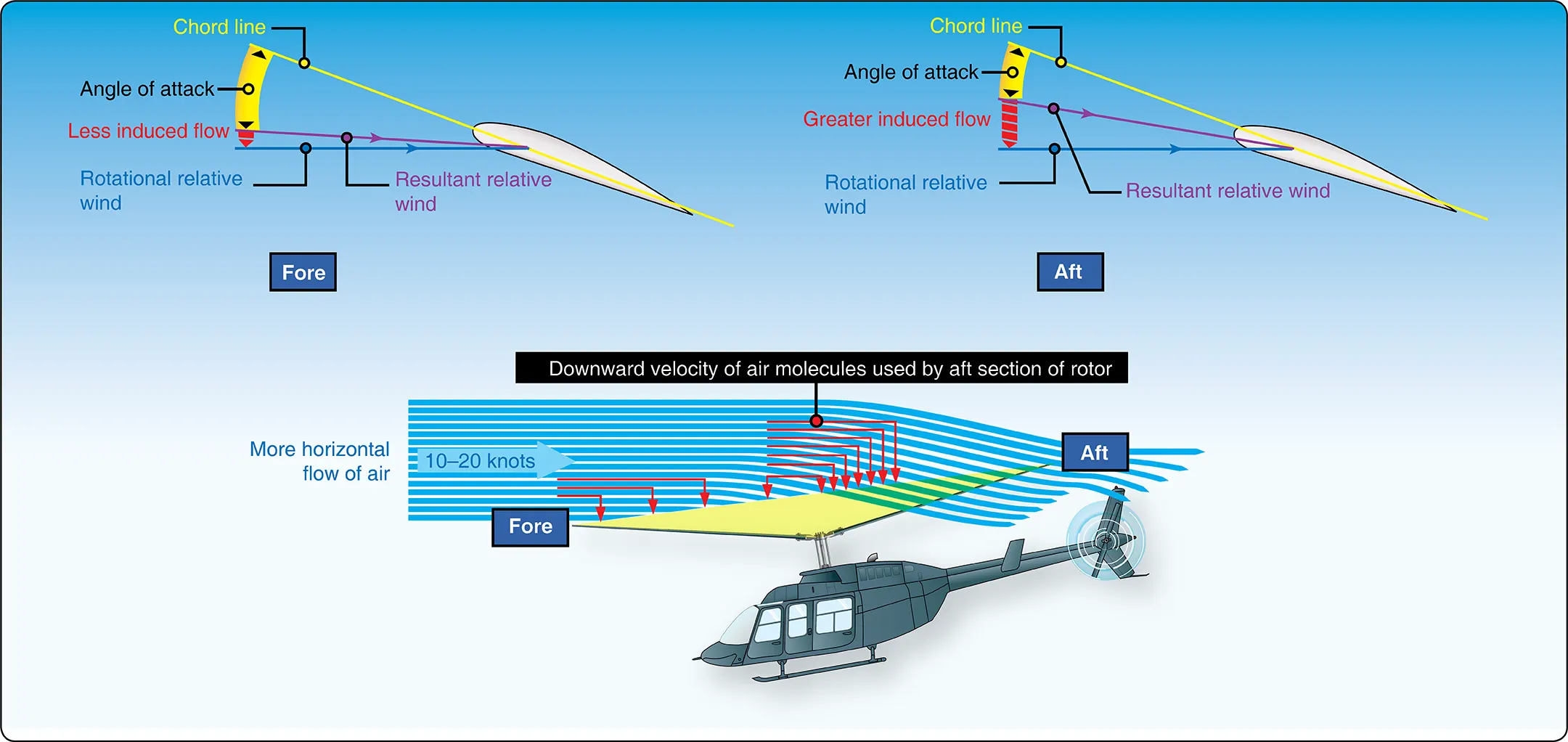
In Ground Effect (IGE)
Ground effect is the increased efficiency of the rotor system caused by interference of the airflow when near the ground. The air pressure or density is increased, which acts to decrease the downward velocity of air. Ground effect permits relative wind to be more horizontal, lift vector to be more vertical, and induced drag to be reduced. These conditions allow the rotor system to be more efficient.
Maximum ground effect is achieved when hovering over smooth hard surfaces. When hovering over surfaces as tall grass, trees, bushes, rough terrain, and water, maximum ground effect is reduced. Rotor efficiency is increased by ground effect to a height of about one rotor diameter (measured from the ground to the rotor disk) for most helicopters. Since the induced flow velocities are decreased, the AOA is increased, which requires a reduced blade pitch angle and a reduction in induced drag. This reduces the power required to hover IGE. [Figure 8]
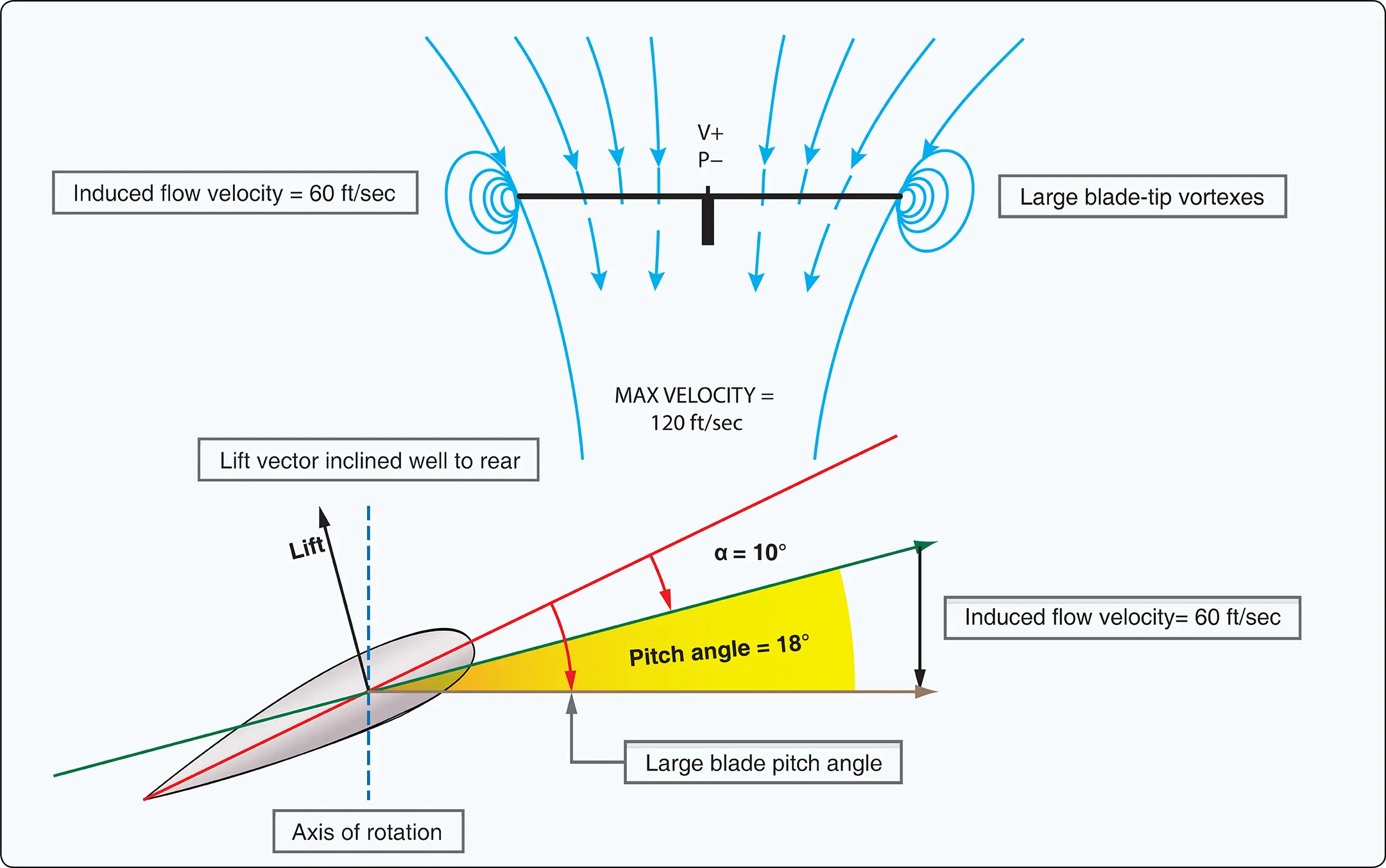
Out of Ground Effect (OGE)
The benefit of placing the helicopter near the ground is lost above IGE altitude. Above this altitude, the power required to hover remains nearly constant, given similar conditions (such as wind). Induced flow velocity is increased, resulting in a decrease in AOA and a decrease in lift. Under the correct circumstances, this downward flow can become so localized that the helicopter and locally disturbed air will sink at alarming rates. This effect is called settling with power. A higher blade pitch angle is required to maintain the same AOA as in IGE hover. The increased pitch angle also creates more drag. This increased pitch angle and drag requires more power to hover OGE than IGE. [Figure 9]
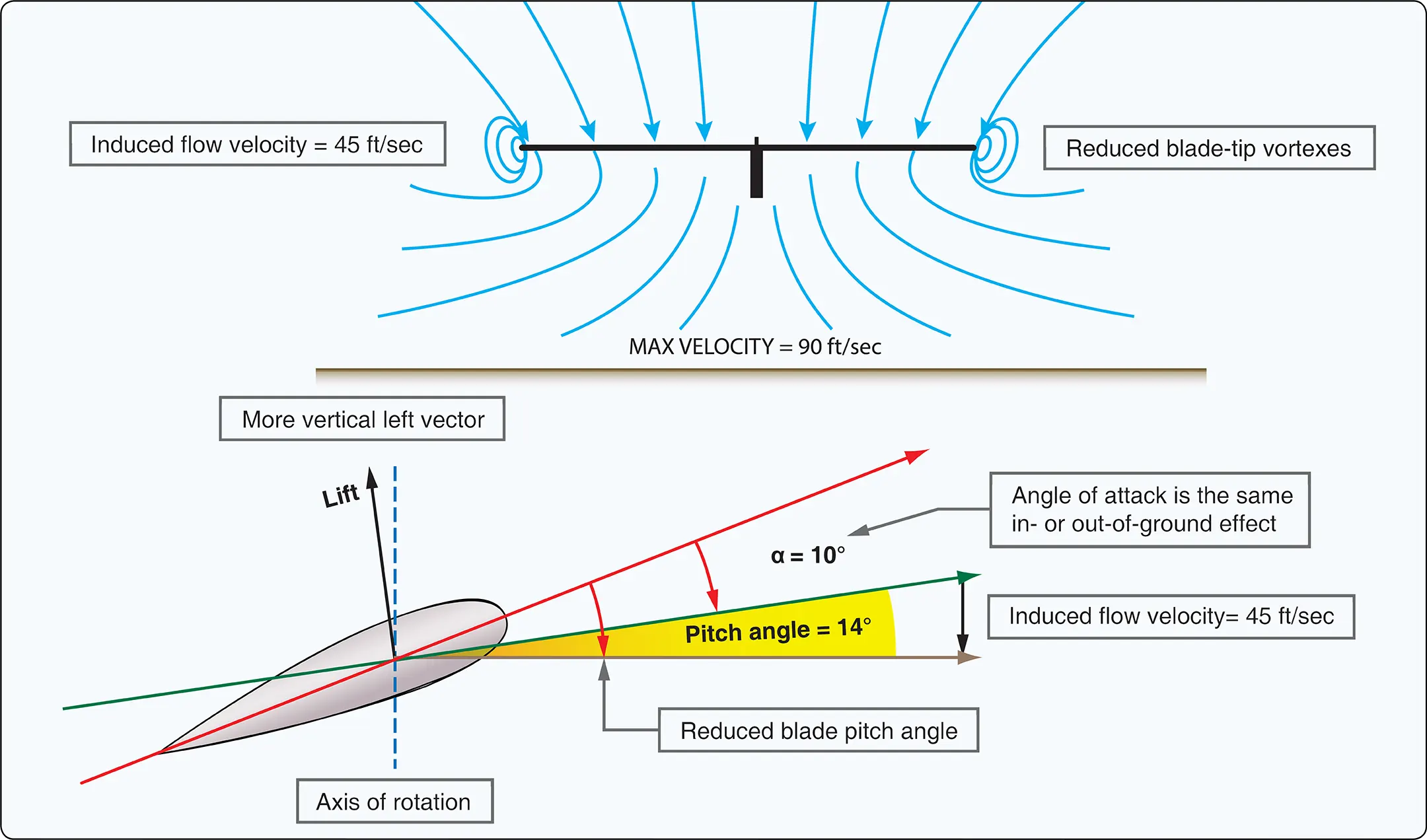
Rotor Blade Angles
There are two angles that enable a rotor system to produce the lift required for a helicopter to fly: angle of incidence and angle of attack.
Angle of Incidence
Angle of incidence is the angle between the chord line of a main or tail rotor blade and the rotor hub. It is a mechanical angle rather than an aerodynamic angle and is sometimes referred to as blade pitch angle. [Figure 10] In the absence of induced flow, AOA and angle of incidence are the same. Whenever induced flow, up flow (inflow), or airspeed modifies the relative wind, the AOA is different from the angle of incidence. Collective input and cyclic feathering change the angle of incidence. A change in the angle of incidence changes the AOA, which changes the coefficient of lift, thereby changing the lift produced by the airfoil.
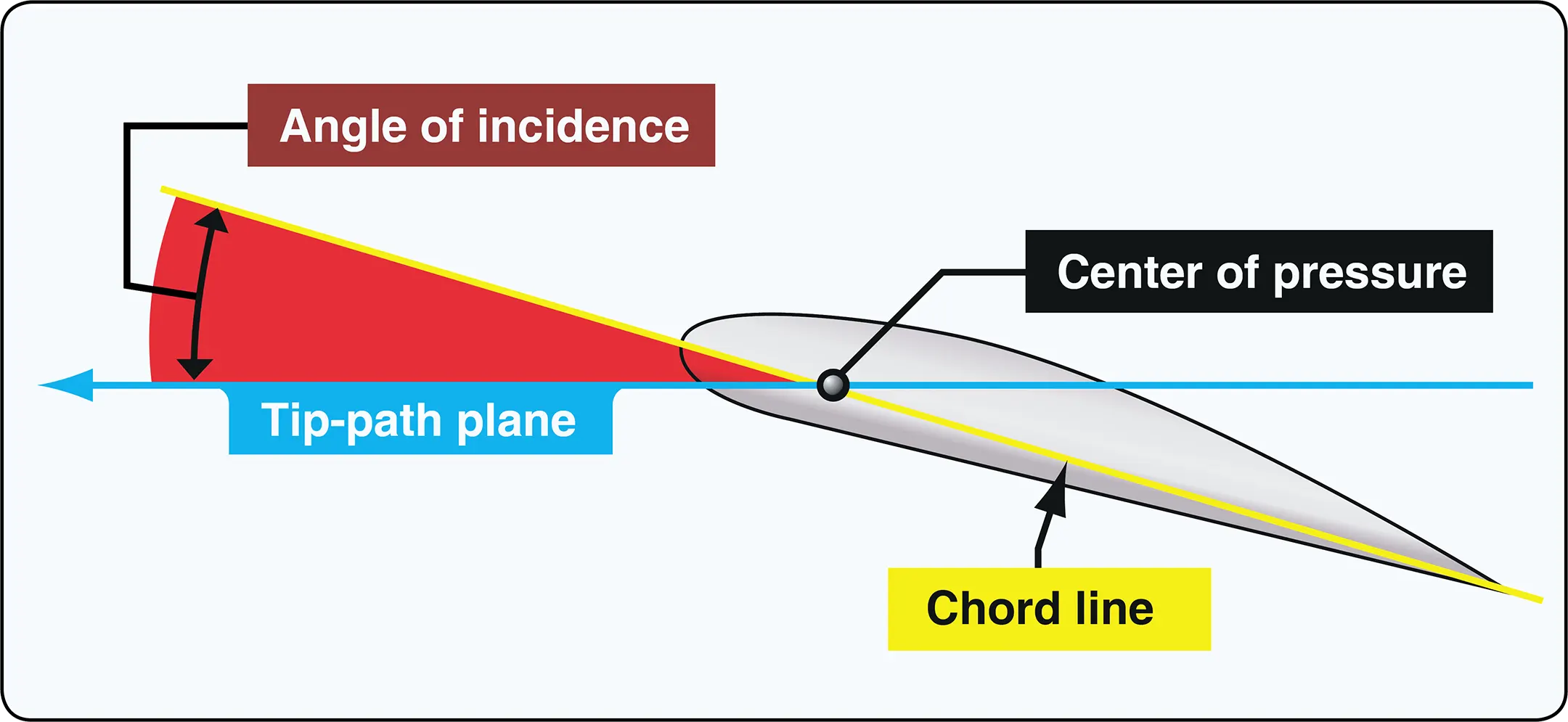
Angle of Attack
AOA is the angle between the airfoil chord line and resultant relative wind. [Figure 11] AOA is an aerodynamic angle and not easy to measure. It can change with no change in the blade pitch angle (angle of incidence, discussed earlier).
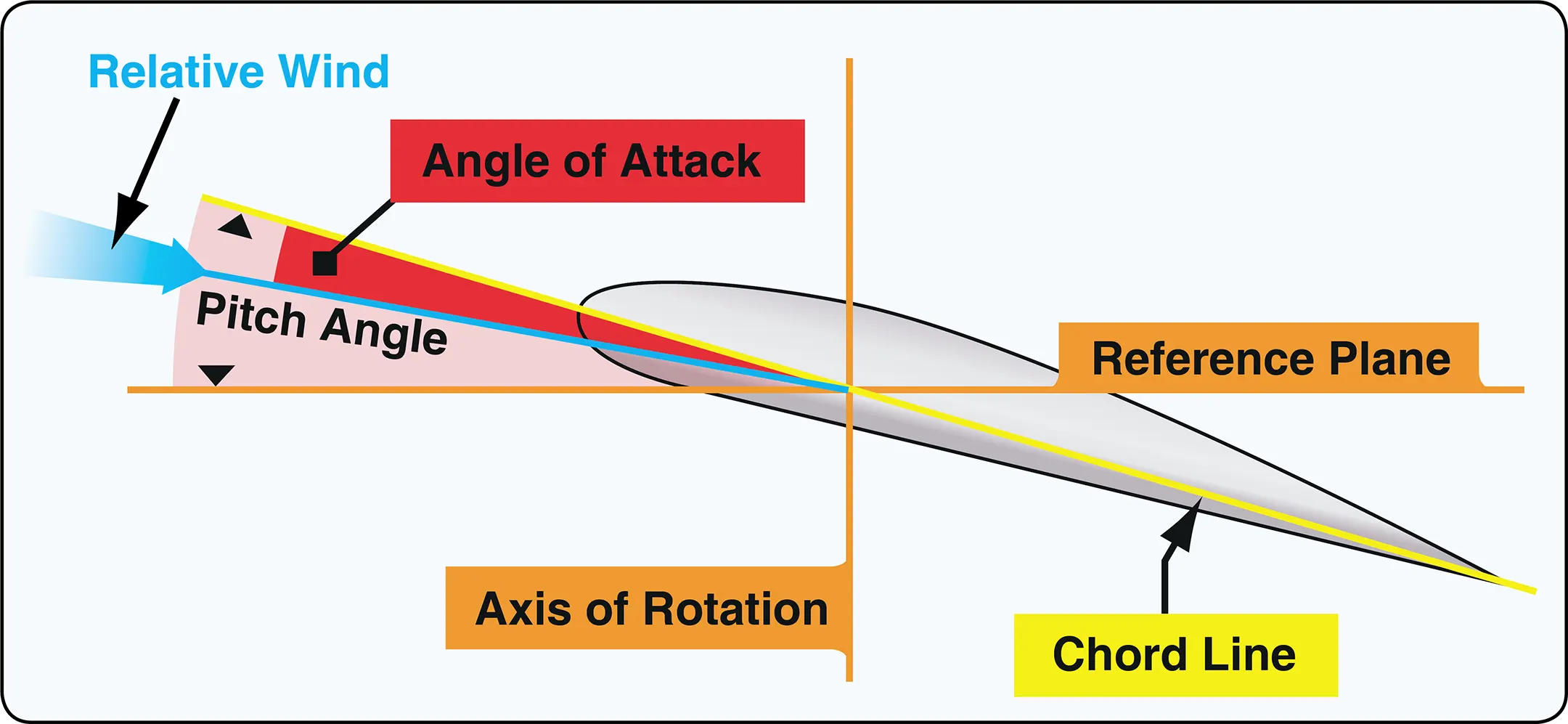
and resultant relative wind
When the AOA is increased, air flowing over the airfoil is diverted over a greater distance, resulting in an increase of air velocity and more lift. As the AOA is increased further, it becomes more difficult for air to flow smoothly across the top of the airfoil. At this point, the airflow begins to separate from the airfoil and enters a burbling or turbulent pattern. The turbulence results in a large increase in drag and loss of lift in the area where it is taking place. Increasing the AOA increases lift until the critical angle of attack is reached. Any increase in the AOA beyond this point produces a stall and a rapid decrease in lift. (refer to the Low Rotor RPM and Rotor Stall post of Helicopter Emergencies and Hazards section)
Several factors may change the rotor blade AOA. The pilot has little direct control over AOA except indirectly through the flight control input. Collective and cyclic feathering help to make these changes. Feathering is the rotation of the blade about its spanwise axis by collective/cyclic inputs causing changes in blade pitch angle. Collective feathering changes angle of incidence equally and in the same direction on all rotor blades simultaneously. This action changes AOA, which changes coefficient of lift (CL), and affects overall lift of the rotor system.
Cyclic feathering changes angle of incidence differentially around the rotor system. Cyclic feathering creates a differential lift in the rotor system by changing the AOA differentially across the rotor system. Aviators use cyclic feathering to control attitude of the rotor system. It is the means to control rearward tilt of the rotor (blowback) caused by flapping action and (along with blade flapping) counteract dissymmetry of lift. Cyclic feathering causes attitude of the rotor disk to change but does not change the amount of lift the rotor system is producing.
Most of the changes in AOA come from change in airspeed and rate of climb or descent; others such as flapping occur automatically due to rotor system design. Flapping is the up and down movement of rotor blades about a hinge on a fully articulated rotor system. The semi-rigid system does not have a hinge but flap as a unit.
The rigid rotor system has no vertical or horizontal hinges so the blades cannot flap or drag, but they can flex. By flexing, the blades themselves compensate for the forces which previously required rugged hinges. It occurs in response to changes in lift due to changing velocity or cyclic feathering. No flapping occurs when the tippath plane is perpendicular to the mast. The flapping action alone, or along with cyclic feathering, controls dissymmetry of lift. Flapping is the primary means of compensating for dissymmetry of lift.
Pilots adjust AOA through normal control manipulation of the pitch angle of the blades. If the pitch angle is increased, the AOA increases; if the pitch angle is reduced, the AOA is reduced.